Research
The Fluid Physics Laboratory focuses on the following research areas:
- Surface Wetting, Electrowetting and Interfacial Science
- Microfluidics and Nanofluidics, Optofluidics and Electrofluidics
- Heat Transfer and Thermal-Fluid Sciences
- Sustainable Energy and Renewable Energy
- Surface Plasmon Resonance and Optical Sensing Technology
- Fluid-based Adaptive, Reconfigurable and Intelligent Electronic Systems
EWOD-Controlled Digital Microfluidics
The electrowetting on dielectric (EWOD) effect has been defined as the change in the contact angle between an electrolyte and a dielectric surface due to an applied electric potential between them. In the presence of control electrodes specially patterned on the substrate, sequential activation of electrode elements can give rise not only to droplet morphing but also to continuous transport on the surface. As a promising technique, EWOD has been applied in various fields such as lab on a chip, adjustable liquid lenses/prisms and electronic displays. In these applications, EWOD allows microdroplets to be independently manipulated under direct electrical control without the use of external pumps, valves or microchannels. Recently, EWOD has drawn tremendous interest in lab-on-a-chip systems due to its programmable and reconfigurable characteristics.
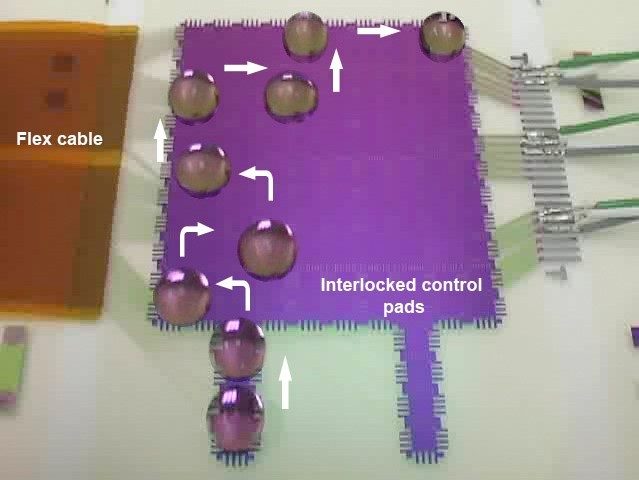
The electrowetting on dielectric (EWOD) effect has been defined as the change in the contact angle between an electrolyte and a dielectric surface due to an applied electric potential between them. In the presence of control electrodes specially patterned on the substrate, sequential activation of electrode elements can give rise not only to droplet morphing but also to continuous transport on the surface. As a promising technique, EWOD has been applied in various fields such as lab on a chip, adjustable liquid lenses/prisms and electronic displays. In these applications, EWOD allows microdroplets to be independently manipulated under direct electrical control without the use of external pumps, valves or microchannels. Recently, EWOD has drawn tremendous interest in lab-on-a-chip systems due to its programmable and reconfigurable characteristics.
High-Heat Flux Electrowetting Cooling with Site-Specific Treatments
We aim to develop an EWOD-based adaptive chip cooling technique with surface treatments. The cooling microdroplets are created from the reservoir (condenser) via EWOD splitting and transported by EWOD through the hydrophobic path to the hot spot with no pumping limitation. A layer of hybrid superhydrophobic lotus-leaf-like textures and superhydrophilic biwick structures is coated on the hot spot surface. The delivered microdroplets will undergo spontaneous self-directed motion towards the hot spot upon contact with the chemically patterned surface with wedge-shaped gradient. The superhydrophilic biwick structures give rise to considerably enlarged meniscus area and significantly promote thin-film evaporation. EWOD-based microdroplet splitting and merging are used to precisely confine microdroplet volumes and hence optimize liquid film thickness. Moreover, capillary instability in an EWOD-driven rivulet enables high-throughput generation of microdroplets and the feedback mechanism of the control system ensures sufficient fluid supply to balance the rate of evaporation on the hot spot.
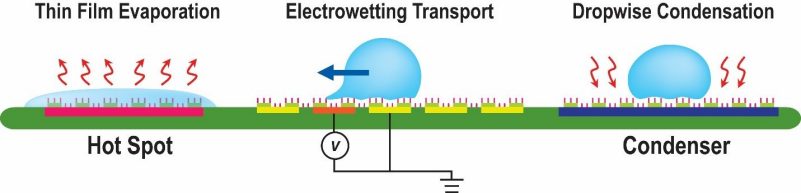
Contact Line Dynamics and Molecular Kinetics Theory
The study of micro/nano-fluidics and wetting-related applications necessitate the insightful knowledge of the physical mechanisms underlying the moving three-phase (solid, liquid and vapor) contact line, which is beyond the predictive capability of the continuum theory of fluid dynamics. In the framework of molecular kinetic theory (MKT), contact line friction (CLF) emerges as a result of the solid-liquid interactions and liquid-liquid interactions, which are termed as solid-liquid retarding and viscous damping, respectively. Solid-liquid retarding is ascribed to the work of adhesion and viscous damping arises from the viscous force exerted on the liquid molecules within the contact zone. In particular, a universal scaling law is being derived by us to describe the CLF on ultrahydrophobic surfaces before the Cassie-to-Wenzel transition.
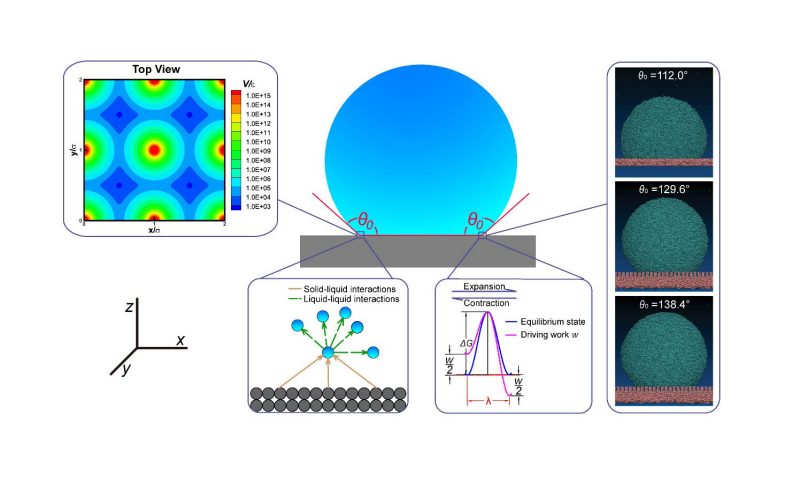
Meridian Whispering Gallery Modes Sensing on Micro/Nanostructured Chip Surfaces
Schematic and operation principles of a sessile microdroplet cavity system on a chip. We put forward to use meridian whispering gallery modes (WGMs) sensing of trace analytes contained in a liquid microdroplet. Coupling light or radiation into the microdroplet is accomplished by placing it on a chip surface that incorporates a planar optical waveguide, along with electrodes that can tune the morphology of the microdroplet cavity via electrowetting to optimize the coupling.
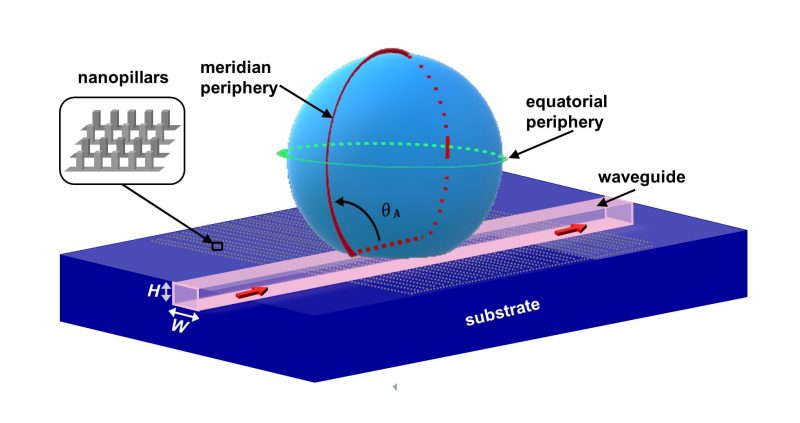
Partial Leidenfrost Evaporation-Assisted SERS Sensing on Hierarchical Plasmonic Micro/Nanostructures
The surface-enhanced Raman spectroscopy (SERS) devices are based on hierarchical plasmonic micro/nanostructures, which are fabricated by implanting nanolaminated metal nanoantennas on carbon nanotubes (CNTs)-decorated Si micropillar arrays. In comparison with natural evaporation, the so-called partial Leidenfrost-assisted evaporation on the hierarchical surfaces can provide a levitating force to maintain the water-based analyte droplet in the Cassie-Wenzel hybrid state, i.e., a Janus droplet. By overcoming the diffusion limit in SERS measurements, the continuous shrinking circumferential rim of the droplet, which is in the Cassie state, towards the pinned central region of the droplet, which is in the Wenzel state, results in a fast concentration of dilute analyte molecules on a significantly reduced footprint within only several minutes. A partial Leidenfrost droplet on the hierarchical plasmonic surfaces can reduce the final deposition footprint of analytes by 3-4 orders of magnitude and enable ultrasensitive SERS detection of nanomolar analytes (10-9 Mole) in an aqueous solution.
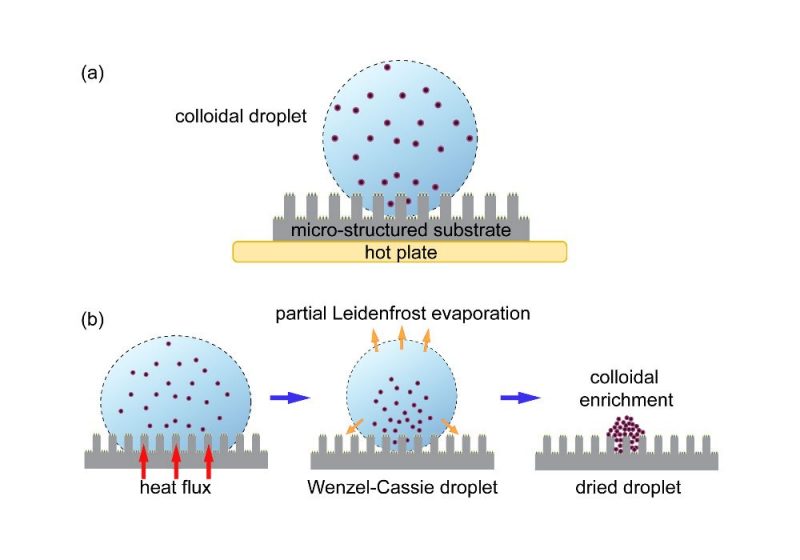
Colloidal Assembly through Holographic Guiding
We have assembled a holographic optical trapping (HOT) system for colloidal assembly purpose. A collimated laser beam (532 nm & 300 mW DPSS laser, Cobolt Inc.) with the output diameter of ~1.2 mm is first expanded by a Keplerian telescope composed of two convex lenses L1 and L2, and is subsequently projected onto a liquid crystal spatial light modulator (SLM) (JD8554 SDK, Japser Display Corp.) The Jasper SLM can generate phase retardation on the wavefront of the incident beam at each 6.4 μm ´ 6.4 μm pixel within a 1920 ´ 1080 array. The SLM is placed between two linear polarizers (P1 and P2) that are adjusted to obtain the desired phase modulation. Then the phase modulated laser beam is further adjusted by the second telescope (L3 and L4) so as to fill the entrance pupil of a high magnification objective lens OL (Nikon 100X/1.25 WD=0.23) mounted on a customized inverted optical microscope. A dichroic mirror (M) reflects the laser light into OL while allowing images of trapped nanoparticles to pass through to a CCD camera.
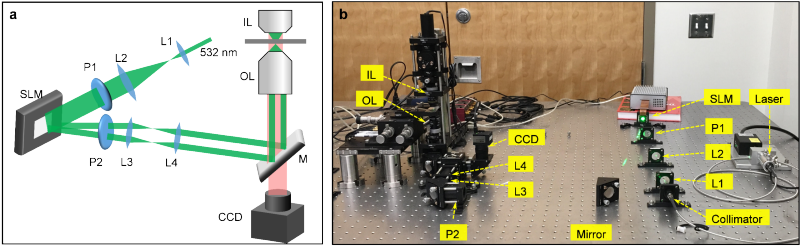